
Hydra vulgaris: The Wnt Signaling Pathway and Preventing Ectopic Head Formation
Stem cell research is a broad topic of interest in the science community, with a range of medical applications including spinal cord and tendon injuries, macular degeneration, pharmacological testing, and rejuvenation [1]. Stem cells are, in general, used in medicine to regenerate damaged mammalian tissue. One important aspect of stem cells is their high activity of the Wnt signaling pathway, which has been studied for over 40 years [2]. Wnt signaling is an integral part of the differentiation of cells in all animals, particularly during embryogenesis but also throughout life, and has been studied in part because of its implication in several cancers [3]. Recent research in cancer treatment has included targeting the Wnt/β-catenin signaling pathway [4]. Additionally, Wnt activators are being used to treat neurodegenerative and metabolic disorders, as well as for anti-aging [5]. However, there are still a lot of unknowns regarding this pathway. It is a complex and difficult pathway to study because there are 19 different Wnt genes in humans and many other proteins and transcription factors involved [6]. In the animal Hydra vulgaris this pathway is active in some capacity at all times and is very active during regeneration [3,7,8,9,10,11]. It is also active during the growth of ectopic heads, which are heads that grow on the wrong part of the Hydra or in addition to an already established head. Hydra is a model organism for studying countless molecular processes due to its structural simplicity and incredible regenerative abilities, including the ability to regrow both halves of a bisected body in just 2 days, and is, therefore, an ideal tool for studying the Wnt signaling pathway [8]. This review will describe new research on the activity of β-catenin, GSK-3β, and general Wnt signaling during regeneration and ectopic head formation and prevention in Hydra.
Background
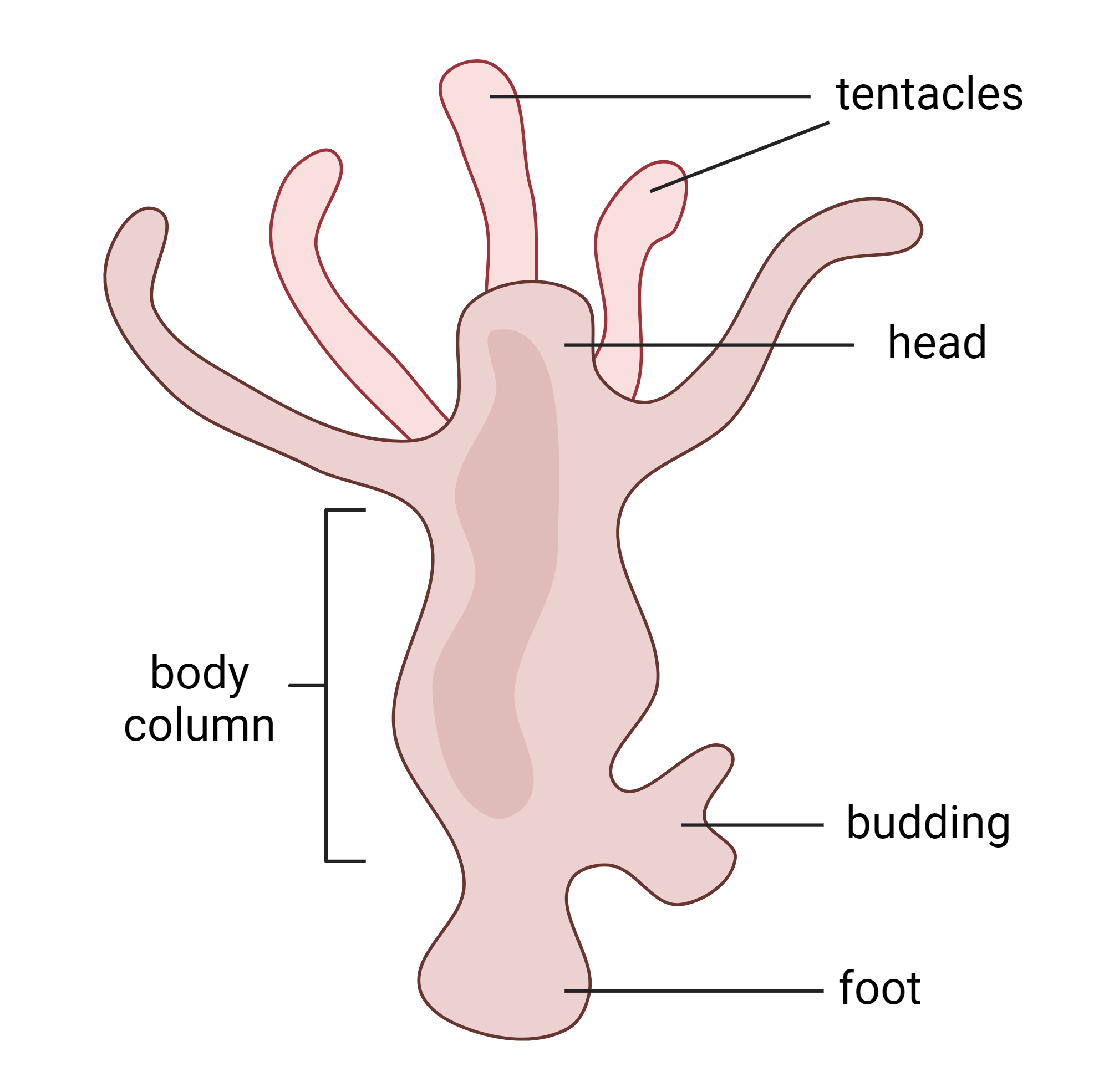
The studies in this review focus on Wnt genes and regulatory components in Hydra vulgaris. The major regulatory proteins of the Wnt pathway are β-catenin (β-cat) and GSK-3β [3,7,8,9,12]. When there is an abundance of β-cat in the cytoplasm, it enters the nucleus and interacts with TCF/LEF transcription factors to transcribe Wnt target genes. GSK-3β acts as a negative regulator of Wnt transcription by degrading β-cat, which prevents β-cat from accumulating enough to enter the nucleus. [3,7,8,9,12]
The model system chosen for these studies is the Hydra, a small animal (about 10-30 mm) that has existed for over 200 million years [13]. Hydra consists of a head and foot connected by a body column. The body is composed of the ectoderm (the outer layer), the endoderm (the inner layer), and interstitial stem cells throughout [3,12]. In the head, there is a head organizer which is comprised of 5-180 cells and is key to regeneration and body axis patterning [3,8,9,10,11,12]. The first sign of ectopic head growth is an ectopic head organizer. β-cat plays a major role in the formation of head organizers, and GSK-3β is important in preventing ectopic head organizers. This is known from experiments where ectopic heads were induced in Hydra after administering an inhibitor of GSK-3β [3,12]. By inhibiting GSK-3β, there was an uptick in β-cat throughout the body column, and this accumulation led to ectopic head formation [3,12]. The more complex mechanisms of preventing ectopic heads are yet unknown.
What is β-cat’s role in head regeneration?
There have been recent advancements in understanding regeneration in Hydra. A useful tool for this research was green fluorescent protein (GFP) which can be attached to many different kinds of proteins and visualizes the localization of that protein under fluorescence microscopy. Using GFP to identify β-cat promoter activity, Iachetta et al. (2018) were able to visualize the relative abundance of β-cat transcription in three lines of transgenic Hydra, with modified expression in either ectodermal, endodermal, or interstitial cells [3]. They followed the fluorescence of each cell type during regeneration for 96 hours post-amputation of the head. There was an increase in β-cat in both interstitial and endodermal cells [3]. These results were expected by the researchers because endodermal cells are key to Hydra regenerative processes, with β-cat target genes highly expressed in endodermal cells and upregulated in the early phase of regeneration. These results also align with the hypothesis that many interstitial cells are able to become the new head organizer before one is established.
According to Petersen et al. (2015), there are three overall steps to Hydra regeneration: early injury response (~0.5h post-amputation), pre-patterning (12h post-amputation), and patterning/differentiation (48-96h post-amputation) [14]. The study by Iachetta et al. (2018) had an unexpected result; their data suggests that β-cat controls or sustains late differentiation programs, as opposed to the previous thought that β-cat is only relevant to the pre-patterning phase [3]. Data presented in a study by Gufler et al. (2018) agrees with the results of Iachetta et al. (2018). Gufler et al. (2018) suggest that β-cat is necessary to advance from the pre-patterning phase to differentiation [9]. In their study, they confirmed that when both the head and foot of a Hydra are cut off the initial pre-patterning response is the same at both sites. Differentiation then occurs when the head and foot are established, and β-cat is an active part of this transition [9].
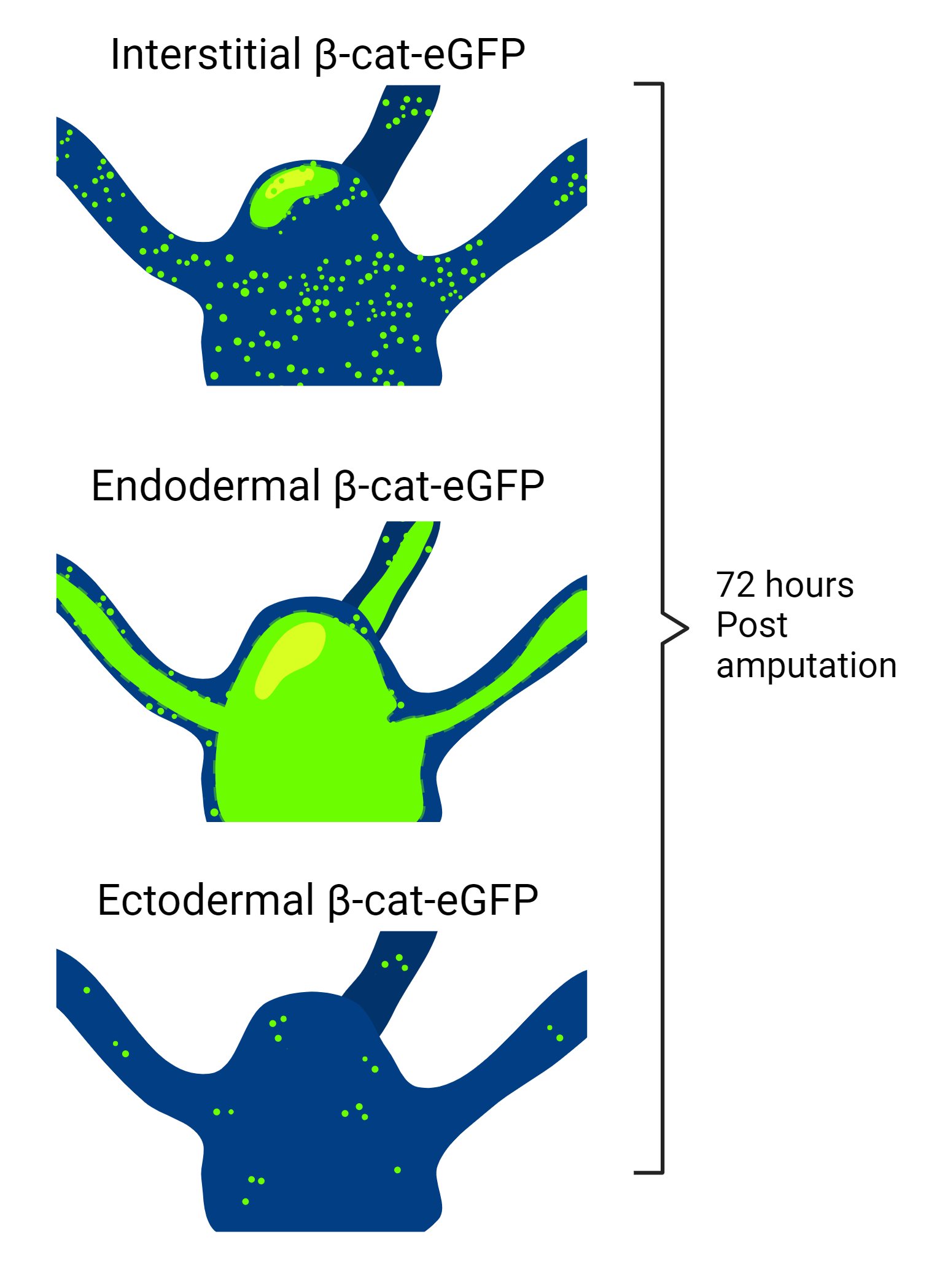
The work by Iachetta et al. (2018) establishes the precise timing of the production of β-cat during regeneration, as well as the type of cell it is produced in, its location in the body, and when it starts to disappear [3]. These results indicate that further transgenic Hydra could be formed using this method with various fluorescent protein markers in order to follow other parts of the Wnt pathway. GSK-3β may be a suitable protein to track during regeneration. Future research could reveal the amount of GSK-3β in cells surrounding the head organizer and down the body column of the Hydra, as well as the timing of its upregulation in these cells. This could be directly compared to the Hydra with tagged β-cat to better understand regeneration and ectopic head prevention during regeneration.
Can a regenerating head organizer prevent ectopic heads?
Head organizers have the intrinsic ability to prevent ectopic heads from forming in a variety of situations. Signals diffuse from the head organizer down the body column in a gradient [12]. One of these signals is a constant inhibitory signal that prevents interstitial stem cells from becoming ectopic head organizers [12]. There is also a constant activator signal that induces the formation of head organizers. This activator is considered a weaker signal than the inhibitor, which is why ectopic heads generally don’t form. When a Hydra head is sliced off, the head organizer is no longer present to send out the inhibitor signal. The activator signal is then able to induce the formation of a new head organizer [3,12]. The exact molecular basis of this activator and inhibitor signaling is still being studied.
It can be hypothesized that the activator signal induces transcription of β-cat and the inhibitor signal induces transcription of GSK-3β. This hypothesis is based on the fact that when GSK-3β is inhibited, β-cat accumulates, and multiple ectopic heads form [3,12]. Interestingly, when both the head and foot of a Hydra are cut off only one head and one foot are regenerated, not multiple [8]. The new head organizer must establish itself very quickly and send inhibitor signals early on in the regeneration process to prevent other interstitial cells from becoming ectopic heads.

Cazet et al. (2021) tested a regenerating head organizer’s ability to inhibit ectopic head formation in different circumstances [8]. They discovered that prolonging the healing of injuries by impaling them for 12 hours caused an increase in Wnt signaling, including at least one head-specific Wnt transcription factor. They predicted that lengthening this “generic injury response” may lead to ectopic head formation because of this irregular increase in Wnt signaling [8]. In the second trial of the experiment, Cazet et al. (2021) cut off both the head and foot of the Hydra, impaled the wounds for 12 hours, and then cut off the slightly regenerated head again before allowing both sites to naturally heal [8]. This ensured the foot regeneration was prolonged and the head regeneration was not. The results were a single ectopic head grown alongside the foot in 61% of cases. Cazet et al. (2021) concluded that lengthening the generic injury response at the foot resulted in abnormal Wnt signaling which led to the ectopic head. However, in the cases where an ectopic head did not grow, Cazet et al. (2021) suggested that the regenerating head organizer had regained its ability to prevent ectopic heads before the ectopic head could establish itself [8]. This implies that the regrowing head organizer may have gained this ability before it was differentiated. More studies need to be done to confirm when during regeneration the head organizer gains the ability to prevent ectopic heads. This should be compared to the timing of the transition from pre-planning to differentiation.
What genes are involved in regeneration and ectopic head prevention?
Recent studies have begun to characterize the genetic basis of head regeneration. Murad et al. (2021) identified promoters and enhancers by their specific “post-translational modifications of histone proteins”, as well as evidence of increased accessibility [11]. 298 genes were identified as being differentially expressed in regeneration and budding (asexual reproduction). Out of these 298 genes, clusters of similarly expressed genes were made (C1-C8) and tracked over 48 hours of head regeneration [11]. The earliest expressed clusters post-amputation were C1, C7, and C5. Cluster C1 was highly expressed from the moment of amputation, but then tapered off to almost no expression within 12 hours [11]. C7 was expressed within 2 hours, and at a higher level than C1. C5 had the strongest expression of any cluster, specifically between 4 and 12 hours post-amputation [11]. These gene clusters can be further analyzed in comparison to the results of other studies.

The timing of expression of these clusters can be applied to the timing of certain events during regeneration. Cazet et al. (2021) identified a generic injury response that can be lengthened to produce abnormal Wnt signaling [8]. This injury response must be the earliest cellular reaction to an injury, identified as the pre-planning phase by Iachetta et al. (2018) and Gufler et al. (2018) [3,9]. It can be assumed that the genes identified in cluster C1 or C7 are responsible for this response because they are the first to activate upon injury. Cazet et al. (2021) also showed that this response can, when lengthened, lead to ectopic head growth alongside the foot [8]. Consequently, C1 or C7 must contain at least some head-forming specific genes.
Cazet et al. (2021) also identified that the regenerating head organizer can prevent ectopic heads very early during regeneration [8]. Cluster C5 may contain the genes responsible for this ability since it was the next cluster expressed and it is expressed at high levels. This would explain why some of the Hydra in the experiment by Cazet et al. (2021) were able to establish ectopic heads while the real head was regenerating, and some were not [8]. There is a time of about 4 hours before C5 is expressed in the regenerating head, which may be the gap that allowed the ectopic heads to establish.
Conclusion
The Wnt signaling pathway is highly active in stem cells in all animals, and studying it in the model system Hydra leads to efficient results because the Hydra regenerates after amputation in just 2 days. The purpose of this review was to describe the specific molecular data recently garnered from this model system. Iachetta et al. (2018) and Gufler et al. (2018) have shown that during regeneration β-cat is not just a major signal to grow a new head, but also an active part of the transition from pre-planning to differentiation [3,9]. Cazet et al. (2021) have shown that the generic injury response in Hydra can be manipulated and controlled to induce ectopic heads [8]. Additionally, Murad et al (2021) have mapped out the genes involved in regeneration so well that, combined with the results of Cazet et al. (2021), it is now known exactly which group of genes is likely responsible for the head organizer having the ability to prevent ectopic heads so early on in regeneration [8,11]. There is a wide range of potential medical advancements from applying this research to human and other animal cell studies, including understanding body mapping mistakes during embryogenesis and the potential to improve healing using regeneration. There is much research to be done to connect the results of this review with human stem cell advancement, starting with comparative studies between the genes identified by Murad et al. (2021) in Hydra with more complex animal genomes [11].

About the Author: Maryann Ferrara
Maryann Ferrara received a Bachelor’s degree in Biochemistry and Molecular Biology in June of 2024. She is interested in becoming a professor and researcher. She works in Dr. Navedo and Dr. Nieves-Cintron’s lab in the pharmacology department at UC Davis. She also earned an English minor because of her interest in writing and literature. She chose to write this paper for her biology writing class after researching the Juliano lab on campus and becoming fascinated by the Hydra. She hopes that further studies of Hydra and its regenerative abilities will advance stem cell-based medicine and increase understanding of embryonic development.
Author's Note
I wrote this literature review for the class Writing in the Sciences: Biology with Professor Amy Goodman-Bide. I chose this topic because of my interest in the Juliano research lab on campus. The Juliano Lab studies Hydra, which I found to be a remarkable creature. When I was researching labs on campus and searching for inspiration, this was the only topic that really caught my eye because of its potential in both genetics and molecular biology. I wanted to pick a topic that was more complex than just testing a new medical treatment. I wanted to strengthen my abilities as a molecular biology student by choosing an involved topic where I had to do a lot of background research before I could begin understanding more recent literature about Hydra. This improved my skills as a researcher and a writer by pushing me to write for an academic audience much more versed in the field than myself. I hope readers will gain an appreciation for this little animal and how important it is for the growing field of stem cell research.
References
Zakrzewski, W., Dobrzyński, M., Szymonowicz, M., & Rybak, Z. 2019. Stem cells: past, present, and future [Internet]. Stem Cell Res Ther. doi:10.1186/s13287-019-1165-5
Reya T, Duncan AW, Ailles L, Domen J, Scherer DC, Willert K, Hintz L, Nusse R, Weissman IL. 2003. A role for Wnt signalling in self-renewal of haematopoietic stem cells [Internet]. Nature. 423(6938):409–414. doi:10.1038/nature01593.
Iachetta R, Ambrosone A, Klimovich A, Wittlieb J, Onorato G, Candeo A, D’andrea C, Intartaglia D, Scotti N, Blasio M, et al. 2018. Real time dynamics of β-catenin expression during Hydra development, regeneration and Wnt signalling activation [Internet]. Int J Dev Biol. 62(4-5):311–318. doi:10.1387/ijdb.180092ct.
Zhang Y, Wang X. 2020. Targeting the Wnt/β-catenin signaling pathway in cancer [Internet]. J Hematol Oncol. 13(1). doi:10.1186/s13045-020-00990-3.
Bonnet C, Brahmbhatt A, Deng SX, Zheng JJ. 2021. Wnt Signaling Activation: Targets and Therapeutic Opportunities for Stem Cell Therapy and Regenerative Medicine [Internet]. RSC Chem Biol. doi:10.1039/D1CB00063B.
Heller RS, Klein T, Ling Z, Heimberg H, Katoh M, Madsen OD, Serup P. 2018. Expression of Wnt, Frizzled, sFRP, and DKK Genes in Adult Human Pancreas [Internet]. Gene Expr. 11(3-4):141–147. doi:10.3727/000000003108749035
Broun M, Gee L, Reinhardt B, Bode HR. 2005. Formation of the head organizer in hydra involves the canonical Wnt pathway [Internet]. Development. 132(12):2907–2916. doi:10.1242/dev.01848.
Cazet JF, Cho A, Juliano CE. 2021. Generic injuries are sufficient to induce ectopic Wnt organizers in Hydra [Internet]. Elife. 10. doi:10.7554/elife.60562.
Gufler S, Artes B, Bielen H, Krainer I, Eder MK, Falschlunger J, Bollmann A, Ostermann T, Valovka T, Hartl M, et al. 2018. β-Catenin acts in a position-independent regeneration response in the simple eumetazoan Hydra [Internet]. Dev Biol. 433(2):310–323. doi:10.1016/j.ydbio.2017.09.005.
Macias-Muñoz A, Mesrop LY, Liang HY, Mortazavi A. 2022. Single cell analysis reveals the molecular signaling and cellular composition of the regenerating Hydra head [Internet]. bioRxiv. doi:10.1101/2022.05.17.492329.
Murad R, Macias-Muñoz A, Wong A, Ma X, Mortazavi A. 2021. Coordinated Gene Expression and Chromatin Regulation during Hydra Head Regeneration [Internet]. Genome Biol Evol. 13(12). doi:10.1093/gbe/evab221.
Bode HR. 2012. The head organizer in Hydra [Internet]. Int J Dev Biol. 56:473–478. doi:10.1387/ijdb.113448hb.
Schwentner M, Bosch TCG. 2015. Revisiting the age, evolutionary history and species level diversity of the genus Hydra (Cnidaria: Hydrozoa) [Internet]. Mol Phylogenet Evol. 91:41–55. doi:10.1016/j.ympev.2015.05.013.
Petersen HO, Höger SK, Looso M, Lengfeld T, Kuhn A, Warnken U, Nishimiya-Fujisawa C, Schnölzer M, Krüger M, Özbek S, Simakov O, Holstein T. 2015. A Comprehensive Transcriptomic and Proteomic Analysis of Hydra Head Regeneration [Internet]. Mol Biol Evol. doi:10.1093/molbev/msv079