
Quantitative Analysis of Frontal Cortical White Matter in Fragile X-Associated Tremor/Ataxia Syndrome Postmortem Brain Tissue
Abstract
Fragile X–Associated Tremor/Ataxia Syndrome (FXTAS) is a late-onset neurodegenerative white matter disease associated with premutation alleles (55<n<200 triple nucleotide CGG expansion repeat) in the fragile x messenger ribonucleoprotein 1 gene (FMR1). Since there is currently no cure for FXTAS, there is a need to elucidate the pathology to aid in developing effective treatments. Brain tissue samples are sourced from patients diagnosed with a FXTAS premutation, as well as from individuals who have no diagnosed illnesses but have all willingly donated their brains for scientific research. Tissue samples of the dorsolateral prefrontal cortex (BA46) were collected from 10 confirmed FMR1 premutation carriers and 10 control non-FMR1 premutation carriers. Each sample was cryoprotected, cut into 14µm sections, and immunohistochemically stained using ionized calcium-binding adapter molecule 1 (IBA-1) to visualize microglia. Slides were developed using DAB and counterstained with hematoxylin. The number of microglia in five of the 10 samples were counted using the Cell Counter function on ImageJ software. Microglia were quantified based on stain type and cellular morphology at 60x on an Olympus brightfield microscope. The FXTAS tissue showed dystrophic morphological differences of microglia in comparison to the microglia in control tissue. Further analysis is needed to quantify the various states of microglia morphology in different regions of the brain.
Faculty-sponsored Research
The research of this article was supervised by Verónica Martínez Cerdeño, Ph.D. at the UC Davis Department of Pathology & Laboratory Medicine
Introduction
Fragile X-associated tremor/ataxia syndrome (FXTAS) is a late-onset neurodegenerative disorder associated with premutation alleles involving a repeated sequence of CGG (cytosine-guanine-guanine) nucleotides. This genetic mutation, consisting of only three nucleotides, results in debilitating motor and cognitive function impairment later in life. In some individuals, this CGG sequence expands beyond the normal range (55<n<200 CGG expansion repeat) in the FMR1 gene [1]. An individual with more than 55 but fewer than 200 CGG repeats in the FMR1 gene, is considered a FXTAS premutation carrier. More than 200 CGG repeats result in Fragile X syndrome. FXTAS was first discovered by UC Davis MIND Institute researcher Dr. Randi Hagerman in 2001; the disease pathology in various areas of the brain have not yet been fully characterized [2].

A larger CGG nucleotide repeat before the promoter of the FMR1 gene results in more mRNA transcription but does not result in the synthesis of more Fragile-X Messenger Ribonucleoprotein (FMRP) (Figure 1). FMRP is critical for regulating mRNA translation during protein synthesis in the dendritic spines of neurons, which is essential for brain development and maturation. The expansion repeat disrupts translation; the greater the quantity of mRNA, the more it sequesters other proteins required for producing sufficient levels of FMRP protein [3]. This has been associated with intranuclear inclusions in neurons and other neuroglia, and iron deposits in the brain that may compromise the integrity of white matter tracts, such as the middle cerebellar peduncles. There are three cerebellar peduncles (upper, middle, and lower), which are structural fibers that connect the pons to the cerebellum [4] and are associated with the following clinical symptoms of FXTAS: intention tremor, gait ataxia, cognitive impairment (difficulties with speech and memory), and autonomic dysfunction [5]. FXTAS pathology is only studied posthumously, but the middle cerebellar peduncle (MCP) sign (Figure 2) is the primary antemortem diagnostic feature associated with FXTAS. MCP sign is often used in combination with genetic testing, symptom tracking, and family history to diagnose an individual with FXTAS [1,5].

Neurons, which predominate in the gray matter of the brain parenchyma, are a type of brain cell. In contrast, non-neuronal brain cell types, known as neuroglial cells, include astrocytes, oligodendrocytes, and microglia. These non-neuronal cells exist throughout all parts of the brain but primarily reside in the white matter of the brain parenchyma. Investigating the importance of microglia in the FXTAS brain starts by identifying which areas of the brain are experiencing changes in morphology and number of microglia. Previous research shows that FXTAS is associated with a state of neuroinflammation in which glial cells play a role [6]. Scientists are working to understand how FXTAS is compromising or changing glial cells, specifically, microglia. Microglia are the resident immune cells and macrophages of the central nervous system (CNS) (Figure 3).

Microglia are highly mobile and phagocytic cells with morphology specific to distinct stages of activation in response to brain injury, illness, and aging (Figure 4) [6,7,8]. In the first stage (Stage 1A) microglia soma are small with ramified and extensive cytoplasmic limb-like projections branching out to survey the brain parenchyma, these projections are called processes [7]. In the second stage (Stage 2A), the soma diameter has increased by 1.5 to 2 times larger than the first stage size [7]. Cell processes have started to retract into the soma and the most proximal processes have thickened. In the third stage (Stage 3A), the soma diameter has increased 2 to 3 times larger, and all processes have thickened and retreated to be closer to the cell body [7]. In the fourth stage (Stage 4A) the soma diameter is 3 to 4 times larger than Stage 1A, there are no more thin processes surveying the parenchyma; they have all retracted and only the thick branches remain close to the soma [7]. In the fifth stage (Stage 5A), the soma has increased 5 times larger than the size of Stage 1A. At this stage, there are no more ramified processes; instead, a single thick process transforms into one thin branch. This branch is distinct from the regular surveying processes, extending from the soma and pointing in the direction of cell movement [7]. Stage 5A is characterized by this single branch often oriented towards a point of injury [7,8]. Once microglia reach the sixth stage (Stage 6A), they become macrophages and are fully active, characterized by an amoeboid cell body with short processes or none at all, and highly motile; hunting for targets to phagocytose [7]. Microglia are constantly shifting between these stages of activation; they do not remain in one stage [7]. Resting microglia (Stage 1A) become active and go through each stage and once they become macrophages (Stage 6A), they phagocytose other cells or cell debris [7]. As macrophages, the soma appears larger than any other stage because it is thought to be multinucleated, possibly due to the cell body holding in other cell nuclei that it consumed (Stage 6R the multinuclear stage) [7]. Microglia constantly go through the 6 stages of activation and 6 stages of returning back to a surveying state (Stage 1A).

The hypothesis is that FXTAS tissue will have different microglia cell counts than non-FXTAS samples in the white matter of the BA46 region. This research attempts to establish a protocol that produces consistent tissue results for visualization and to elucidate the relationship between microglia and neurodegeneration because there is some debate as to whether microglial activation initiates neurodegeneration, or if it is the result of neurodegeneration [6].
Methodology
Brain samples were procured from the CENE brain bank after individuals consented to donate their tissue. It is widely observed that brain cells do not necessarily undergo immediate cell death after somatic death. Therefore, the post-mortem interval—the time between death and fixation of brain tissue—is critical for postmortem histological analysis of cells. All tissue samples were provided by males, five from confirmed FMR1 premutation carriers (69 ± 7 years) and five male control non-FMR1 premutation carriers (62 ± 12 years). Brodmann area 46 (BA46), otherwise known as the dorsolateral prefrontal cortex (DLPFC), was chosen for examination because it is an area that has been associated with memory, movement, and planning, which FXTAS negatively impacts (Figure 5) [9].

All sample brains were anatomically cut and the BA46 region of the DLPFC was sectioned from each sample. Each piece was cryoprotected and embedded in Optimal Cutting Temperature (OCT) medium. Thin 14µm slices were cut from the OCT block using a cryostat (Figure 6) and carefully adhered onto slides labeled with the Brodmann region, the sample identity number, and the slide number. Each slide was immunohistochemically stained using ionized calcium-binding adapter molecule 1 antibody (IBA-1) to bind and visualize microglia for detection on slides. Slides were developed using nickel DAB which enhances color sensitivity and counterstained with hematoxylin to provide the background contrast for the other cell colors. After the slides were refrigerated and the coverslips set, they were imaged at 60x on an Olympus brightfield microscope. Lastly, 1mm2 x 0.5mm2 cell count boxes were digitally placed on the white matter of the slides and ImageJ software was used to count the number of microglia. Microglia were quantified based on the presence of a visibly dark soma and points of centrality where processes extended from.

Results
Immunohistochemical staining of the slides using IBA-1 colors the microglia with a brown pigment, allowing us to quantify microglia based on the presence of a visibly dark soma—the cell body. The soma serves as a point of centrality from which cytoplasmic processes extend. Microglia that did not follow this criterion were characterized as dystrophic, implying that the microglia cannot properly engage in the phagocytic removal of dead or decaying cells.


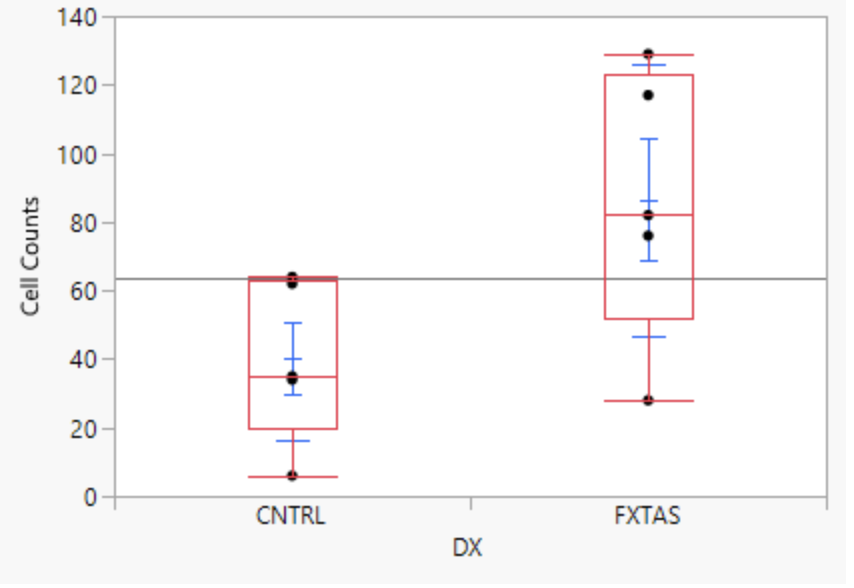
Discussion
The microglia in the control samples displayed darker, rounder, and larger soma with processes in various states of extension and are still attached to their cell bodies, which may suggest that they can progress through stages of activation, from surveying the brain parenchyma to consuming cellular waste and returning to a resting state. The FXTAS samples often exhibited microglia bodies that were small or stretched, with gnarled processes. Some of these processes appeared to be fragmented or completely detached from the cell body [6]. Both FXTAS and control samples showed signs of senescent (aging) microglia which appeared as beaded and pili processes, but the high number of processes detached from the cell body in the FXTAS samples indicate a more advanced stage of senescence in the microglia. This is concerning because microglia are the first responders when pathological changes occur. In the presence of pro-inflammatory cytokines such as interleukin-1β (IL-1β) and tumor necrosis factor-alpha (TNF-α), and the absence of anti-inflammatory cytokines, microglial cells convert to M1 phenotype and secrete more pro-inflammatory factors and reactive oxygen, iron, and nitrogen species. These free radicals perpetuate inflammatory responses, inducing neurotoxicity affecting the entire brain and could be contributing to neuroinflammation and disruption of cellular processes resulting in deterioration of the brain parenchyma [6,8].
Another observation of the sample tissue showed microglia surrounding blood vessels in the brain. In all five FXTAS samples, microglia had morphed around the blood vessels which were not present in the controls. In control tissue, microglia could be proximal to the blood vessels or appear to be lying on top of the vessels, but they were not changing their shape to interact with the blood vessels themselves. Microglia are the only neuroglial cells derived from the embryonic mesoderm which gives rise to cells of the blood and immune system; thus, they are always in proximity to vasculature and are crucial to blood-brain barrier integrity [10]. It is unclear; however, one way microglia could contribute to neurodegeneration is through the phagocytosis—eating away—of endothelial cells that comprise the blood vessels’ outer lining. In addition, microglia may have a unique sensitivity to the post-mortem interval (PMI) because, as the brain ceases function, these first responders may sense and react abnormally to the diminishing oxygen levels, which may explain why they surround vasculature.
On average, individuals with FXTAS had twice the number of microglia in the white matter of their DLPFC compared to controls. The microglia in FXTAS samples appear dystrophic, potentially compromising their cellular functions. The prevalence of dystrophic microglia in the FXTAS brain may suggest a neuroinflammatory pathogenesis mediated by these cells. Given the highly dystrophic nature of the microglia observed, it is premature to determine whether their presence in specific brain regions contributes to disease pathology. Moreover, it remains uncertain if the microglial activation seen in FXTAS cases initiates neurodegeneration, as the cells may be incapable of performing any pro- or anti-inflammatory activities.
The hypothesis is not supported by the preliminary cell count data, but the small sample size and a more discriminatory microglia cell count criterion warrants further analysis. Future directions of research include determining microglia stage type, morphology, localization, as well as examination of differences in microglia across gender, age, and severity of disease to see if anti-inflammatory treatments can be used to slow FXTAS pathology and alleviate symptoms.

About the Author: La Rissa Vasquez
La Rissa was a class of 2024 Neurobiology, Physiology, & Behavior major with a minor in Professional Writing. She was an Editor and Scientific Illustrator for the Aggie Transcript and enjoys doing art and listening to music in her spare time.
About the Author: Y. Belle McLennan
Y. Belle McLennan is a graduate student at the Graduate Group in Integrative Pathobiology.
About the Author: Forrest McKenzie, M.D.
Forrest McKenzie, M.D. is a researcher at the UC Davis MIND Institute.
References
- Hagerman RJ, Hagerman P. 2016. Fragile X-associated tremor/ataxia syndrome — features, mechanisms and management. Nature Reviews Neurology. 12(7):403–412. doi:10.1038/nrneurol.2016.82.
- Hagerman RJ, Leehey M, Heinrichs W, Tassone F, Wilson R, Hills J, Grigsby J, Gage B, Hagerman PJ. 2001. Intention Tremor, Parkinsonism, and Generalized Brain Atrophy in Male Carriers of Fragile X. Neurology. 57(1):127–130. doi:10.1212/wnl.57.1.127.
- Berman RF, Buijsen RA, Usdin K, Pintado E, Kooy F, Pretto D, Pessah IN, Nelson DL, Zalewski Z, Charlet-Bergeurand N, et al. 2014. Mouse models of the fragile X premutation and fragile X-associated tremor/ataxia syndrome. Journal of Neurodevelopmental Disorders. 6:25. doi:10.1186/1866-1955-6-25.
- Patestas MA, Gartner LP. 2016. Cerebellum. In: A Textbook of Neuroanatomy (2nd ed.). Hoboken (NJ): Wiley-Blackwell. 279–302.
- Gaillard F, Campos A, Hacking C, et al. Fragile X-associated tremor/ataxia syndrome. Reference article, Radiopaedia.org. Accessed December 5, 2023. Accessed from: https://doi.org/10.53347/rID-1359.
- Martínez Cerdeño V, Hong T, Amina S, Lechpammer M, Ariza J, Tassone F, Noctor SC, Hagerman P, Hagerman R. 2018. Microglial cell activation and senescence are characteristic of the pathology fxtas. Movement Disorders. 33(12):1887–1894. doi:10.1002/mds.27553
- Jonas RA, Yuan T-F, Liang Y-X, Jonas JB, Tay DKC, Ellis-Behnke RG. 2012. The Spider Effect: Morphological and Orienting Classification of Microglia in Response to Stimuli in Vivo. PLoS ONE. 7(2):e30763. doi:10.1371/journal.pone.0030763.
- Lively S, Schlichter LC. 2018. Microglia responses to pro-inflammatory stimuli (LPS, IFNΓ+TNFα) and reprogramming by resolving cytokines (IL-4, IL-10). Frontiers in Cellular Neuroscience. 12:215. doi:10.3389/fncel.2018.00215.
- Kolb B, Whishaw IQ. 2010. Organization of the Nervous System. In: Fundamentals of Human Neuropsychology (6th ed.). Johanneshov: TPB. 75–77.
- Rodgers K. Microglia. Encyclopædia Britannica. Accessed December 5, 2023. Available from: https://www.britannica.com/science/microglia.