
Phytophthora ramorum: Transmission, Adaptability, and Management
Introduction
Phytopthora ramorum (P. ramorum), more commonly known as Sudden Oak Death, is a fungal-like oomycete water mold pathogen derived from Asia. P. ramorum affects over 130 botanical species globally [1, 6]. The establishment of P. ramorum in native forest ecosystems raises concerns as it creates a global cascade of ecological impacts, affecting botanical reproduction in nurseries, globally conserved gardens, and food webs across forested ecosystems [6, 7]. P. ramorum creates habitat loss as it spreads and diminishes forests. Each ecosystem is affected independently by the NA1, NA2, EU1, and EU2 lineages [1]. These lineages are geographically separated by two mating types: A1 and A2 [1, 11]. Asexual reproduction in both the A1 and A2 mating types is a result of allopatric speciation [7]. The lack of sexual reproduction between mating types leads to an asexual predominant lifestyle which has also given rise to asexual mutation mechanisms. The process of genetic mutation has led to new levels of gene copies within zoospores [2, 16]. These rapidly evolving mutations throughout P. ramorum’s lineages prevent successful treatment strategies. This review will examine P. ramorum’s transmission and genetic adaptability, as well as treatment strategies against both P. ramorum and its host species.
Transmission and Appearance
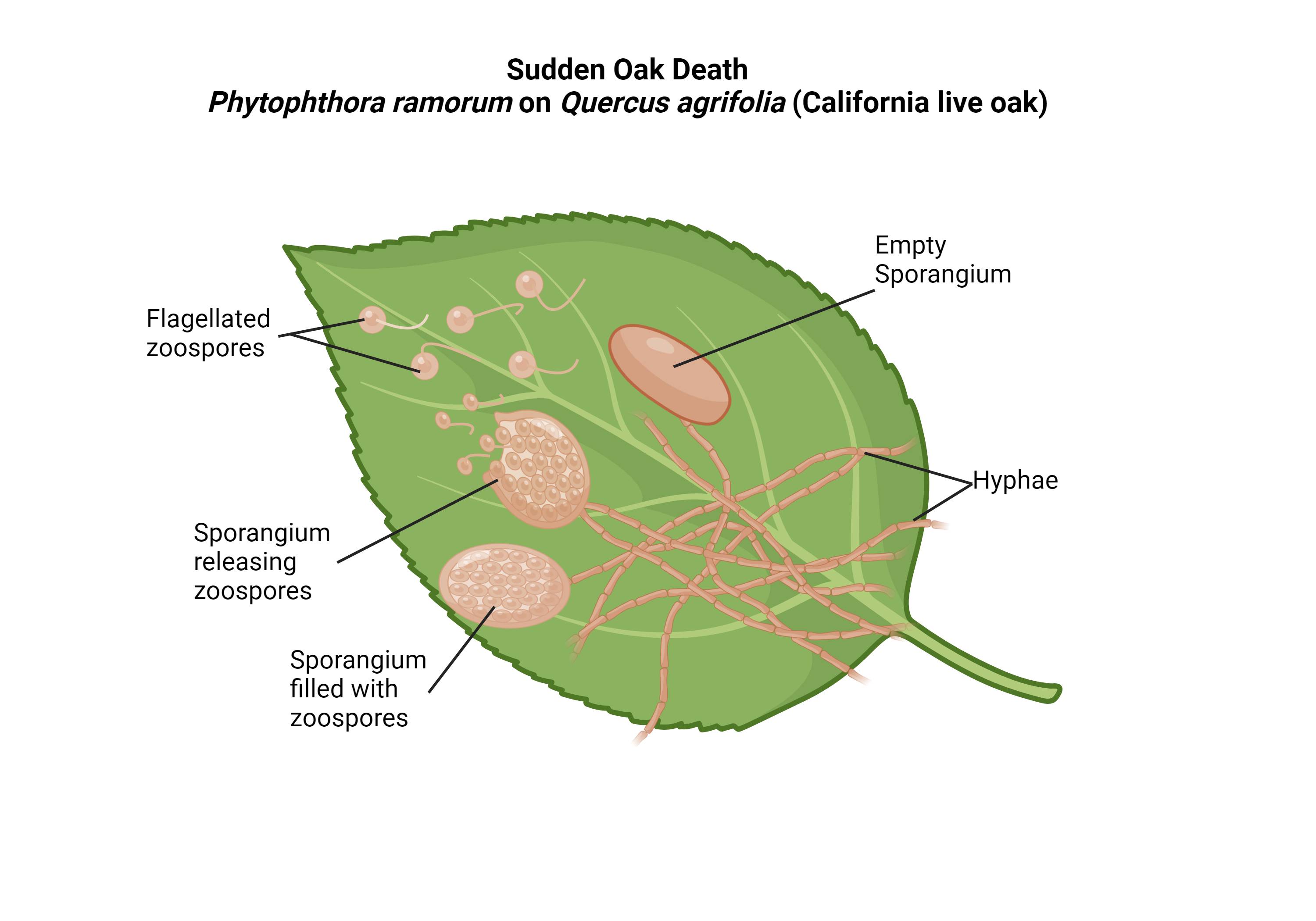
High levels of precipitation, the presence of flagella, and the motility of zoospores facilitate P. ramorum’s transmission throughout the rainy seasons [13]. During the winter months, P. ramorum produces bi-flagellated zoospores (motile asexual spores) that travel readily in wet environments [17]. Windblown rain disperses the bi-flagellated zoospores away from parental colonies, and into the soil where they establish in the roots of host species [4, 13]. Additionally, P. ramorum is transmitted long distances through runoff [12]. In vivo aqueous samples from Marin County, CA were analyzed by McCartney et al. (2018) to determine the presence of zoospores. McCartney et al. (2018) sequenced the samples and found fatty acid residues, which matched the known composition of P. ramorum’s zoospores, further validating the dispersal mechanism of P. ramorum [12]. By moving through soils and hosts, P. ramorum implants zoospores to continue to grow, develop, and spread further diminishing forests throughout the following months.
Following transmission, the success of P. ramorum’s growth is closely correlated with summer daylight periods and average temperatures [15]. Temperature is a key factor in P. ramorum’s development, directly influencing growth rates. As reported in a study conducted by Schweigkofler et al. in 2022, the flexibility of P. ramorum’s development is demonstrated through its wide growth-tolerance brackets which range from 0℃ to 30℃. Conversely, Schweigkofler et al. (2022) and McCartney et al. (2018) conclude that only a narrow range of temperature and diurnal light ideally benefit the growth of P. ramorum. P. ramorum’s optimal growth rate, in which P. ramorum maximizes zoospore production, is between 20℃ and 25℃ [5, 13]. Furthermore, diurnal effects, specifically UV exposure, increase P. ramorum’s growth rate [15]. Moreover, excess UV exposure also serves to aid in the accumulation of genetic mutations that allow this pathogen to evolve [13, 15]. The activity of P. ramorum fluctuates with seasonal changes in rain, temperature, and light. The pathogen's growth and success, despite its sensitivities, reveal its ability to rapidly mutate and adapt.
Adaptability

The allopatric speciation of P. ramorum’s two mating types, A1 and A2, limit the mechanisms by which this pathogen can reproduce. Studies show that P. ramorum has alternative mechanisms to aid in its ability to adapt and reproduce asexually. Dale et al. (2019) examined the reproductive patterns of P. ramorum, noting its limitation to asexual reproduction and the consequential accumulation of rapid beneficial mutations that are difficult to repress [2]. These mutational characteristics that heighten asexual genetic diversity within the A1 / A2 mating types are caused by a loss of heterozygosity (LOH), also known as runs of homozygosity (ROH) [9]. ROH are caused by amino acid malfunctions which change allele frequency. Changes in allele frequencies removes chromosomal heterozygosity, a factor responsible for maintaining genetic diversity, which allows these mating types to gain beneficial recessive genes and maintain them in following generations [2]. Homozygosity is seen to create diversity in P. ramorum due to the expression of hidden recessive traits, which might have otherwise been invisible to selection within heterozygotic individuals [2]. The loss of genetic heterozygosity through ROH is found in repeating scaffolds within P. ramorum’s genome [2, 9]. Mutation patterns among P. ramorum’s lineages, as a result of asexual reproduction, indicate differentiation in protein-coding sequences between mating types. Furthermore, Dale et al. (2019), Elliott et al. (2018), and Kasuga et al. (2018) discovered that resulting adaptations create weak interactions between the DNA base-pairs of adenine and thymine, forming breakpoints in the genome [2, 3, 9]. Chromosomal breakpoints serve as locations for the insertion of transposable elements (TEs), a type of mobile DNA, creating new genetic combinations and often resulting in beneficial mutations [2, 3]. The mutations induce genetic evolution, driving the global spread and infection of P. ramorum. Future research should examine the potential of sexual recombination as a result of allopatric species rejoining. To prevent optimal recombination via sexual recombination, management against P. ramorum is critical in preventing the spread and evolution of this pathogen.
Management
P. ramorum is a steadily evolving pathogen whose multiple lineages pose difficulties in repressing growth. Chemical fungicidal agents, such as phosphite, have been most successful when managing the pathogen. Solla et al. (2021) investigated the most efficient mechanism to introduce phosphite into the environment. The introduction of phosphite fungicides typically occurs via leaf, trunk, or aerial spray methods [14]. Furthermore, Hunter et al. (2018) examined the subsequent internal effects of aerial phosphite implementation within both P. ramorum and their host species. The onset of phosphite, and the mechanism of their implementation, indirectly impacts P. ramorum by demonstrating an upregulation in the innate immunity of host species responsible for the detection of diseases and pathogens [10]. Fungicides, such as phosphite, also increase detoxifying mechanisms in the pathogen’s genome, which work to remove the harmful effects caused by phosphite [10]. Kasuga et al. (2021) examined an increase in enzymatic secretion in host species via the upregulation of genes in the innate immune system to prevent the infection of P. ramorum. Pathways in the innate immune system induced by phosphite treatment include phenylpropanoid biosynthesis and phenylalanine metabolism [10]. These two enzymatic pathways function to increase the genetic dosage of phenylalanine ammonia-lyase (PAL) enzymes [10]. PAL upregulates the expression of antimicrobial agents and lignin concentration in the host’s tissues [10, 18]. The added regulation of microbial agents increases disease surveillance while lignin increases the density of bark and wood to slow the spread of P. ramorum. Together, these processes are then followed by additional regulation via receptor-like serine/threonine kinase (RLKs) [10]. RLKs regulate the transportation of microbes, which helps host species detect the invasion of P. ramorum in living cells [18]. The hosts’ immune system increases defensive enzymes to control the invasion of P. ramorum in the presence of phosphite, suppressing the pathogen, and aiding in preventing P. ramorum’s spread .
As phosphite acts to aid host species, this chemical also has an effect on the metabolism of P. ramorum. Hunter et al. (2018) examined how phosphite treatment is an effective agent against the spread of P. ramorum because it directly suppresses the pathogen’s growth rate [8]. The toxic effect of phosphite alters the vitamin B6 biosynthesis and ionic transport pathways in P. ramorum [10]. The genes involved in P. ramorum’s vitamin B6 biosynthesis serve as a detoxifying agent; they become upregulated as a response to increased cell death upon the toxic onset of phosphite into the pathogen’s cellular physiology [8, 10]. Furthermore, cellular membrane proteins, such as ionic ATP-binding cassette membrane transport proteins, increase in an effort to remove toxins from P. ramorum’s cells [10]. As energy expenditure is focused on the removal of phosphite toxins, P. ramorum’s growth is suppressed during the implementation stages of phosphite treatment due to an increase of toxic elements. Phosphite has also proved not to discriminate between the diverse lineages and growth rates of the pathogen. P. ramorum’s EU and NA lineages, residing in Europe and the United States respectively, indicate similar changes in gene dosage to compensate for higher toxicity levels when phosphite is applied. Although the effects of phosphite against P. ramorum are successful, overuse of this management strategy can increase P. ramorum’s tolerance to these treatments [8, 10]. Modified use of phosphite treatment and the development of alternative management strategies in forests is the first step towards removing P. ramorum.
Conclusion
Recent literature has demonstrated the mechanisms in which P. ramorum has evolved and spread globally. It is evident that pathogenic transmission success is correlated with the reproductive processes and genetic adaptations of P. ramorum. The evolution of these adaptations continues to provide a range of mechanisms for the pathogen to overcome environmental hardships. The frequency of these mutations complicates P. ramorum’s management strategies. Future studies should examine the potential impacts of a sexual hybrid, as P. ramorum’s transmission out of nurseries and into native environments increases the probability of a sexual hybrid developing. However, as of now, significant evidence suggests that phosphite can battle the growth of P. ramorum. Fungicidal treatments suppress the growth and infection of P. ramorum in host species. Although the reception to phosphite in hosts and the pathogen are effective, the success is limited by the possibility of increased tolerance levels due to over-implementation. Future studies will likely focus on the genetic aspects that contribute to the evolution of P. ramorum while investigating more efficacious treatment methods.

About the Author: Kaitlyn Keshishian
Growing up along the Northern California coastline, I have witnessed the heart-wrenching devastation brought to our forests by Pytophthora ramorum, commonly known as Sudden Oak Death. Comprehending this insidious, “invisible” pathogen has become a topic of importance to me. The towering redwoods and oaks that define our landscape are not just trees but the foundation of our ecosystem and history. To protect this precious natural world and the countless species it supports, I am dedicated to raising awareness of the dire need to study and understand P. ramorum’s effect on our environment. P. ramorum is not merely an ecological issue; it's a call to protect our coastal heritage and the future of our forests for future generations.
Author's Note
This review was written for my UWP 102B class, and is targeted for a scientific audience. I wrote this piece because I live along the coastline of Northern California, where P. ramorum, also known as Sudden Oak Death, is invading. Living in a region where this pathogen is so prominent, I wanted to learn more about the current research and treatment strategies. I hope, as a reader, you are able to gain insight and learn more about a significant environmental issue that is not publicly discussed, yet exists across the globe.
References
Dobb RC, Haas SE, Kruskamp N, Dillon WW, Swiecki TJ, Rizzo DM, Frankel SJ, Meentemeyer RK. The magnitude of regional‐scale tree mortality caused by the invasive pathogen Phytophthora ramorum. Earth’s Future. 2020;8(7). http://dx.doi.org/10.1029/2020ef001500. doi:10.1029/2020ef001500
Dale AL, Feau N, Everhart SE, Dhillon B, Wong B, Sheppard J, Bilodeau GJ, Brar A, Tabima JF, Shen D, et al. Mitotic recombination and rapid genome evolution in the invasive forest pathogen Phytophthora ramorum. mBio. 2019;10(2). http://dx.doi.org/10.1128/mBio.02452-18. doi:10.1128/mBio.02452-18
Elliott M, Yuzon J, Malar M, Tripathy S, Bui M, Chastagner GA, Coats K, Rizzo DM, Garbelotto M, Kasuga T. Characterization of phenotypic variation and genome aberrations observed among Phytophthora ramorum isolates from diverse hosts. BMC Genomics. 2018;19(1). http://dx.doi.org/10.1186/s12864-018-4709-7. doi:10.1186/s12864-018-4709-7
Fang Y, Coelho MA, Shu H, Schotanus K, Thimmappa BC, Yadav V, Chen H, Malc EP, Wang J, Mieczkowski PA, et al. Long transposon-rich centromeres in an oomycete reveal divergence of centromere features in Stramenopila-Alveolata-Rhizaria lineages. PLoS Genetics. 2020;16(3):e1008646. http://dx.doi.org/10.1371/journal.pgen.1008646. doi:10.1371/journal.pgen.1008646
Garbelotto M, Schmidt D, Popenuck T. Pathogenicity and infectivity of Phytophthora ramorum vary depending on host species, infected plant part, inoculum potential, pathogen genotype, and temperature. Plant Pathology. 2021;70(2):287–304. http://dx.doi.org/10.1111/ppa.13297. doi:10.1111/ppa.13297
Grünwald NJ, LeBoldus JM, Hamelin RC. Ecology and evolution of the sudden oak death pathogen Phytophthora ramorum. Annual Review of Phytopathology. 2019;57(1):301–321. http://dx.doi.org/10.1146/annurev-phyto-082718-100117. doi:10.1146/annurev-phyto-082718-100117
Hamelin RC, Bilodeau GJ, Heinzelmann R, Hrywkiw K, Capron A, Dort E, Dale AL, Giroux E, Kus S, Carleson NC, et al. Genomic biosurveillance detects a sexual hybrid in the sudden oak death pathogen. Communications Biology. 2022 [accessed 2023 May 14];5(1):477. https://www.nature.com/articles/s42003-022-03394-w. doi:10.1038/s42003-022-03394-w
Hunter S, Williams N, McDougal R, Scott P, Garbelotto M. Evidence for rapid adaptive evolution of tolerance to chemical treatments in Phytophthora species and its practical implications. PloS ONE. 2018;13(12):e0208961. http://dx.doi.org/10.1371/journal.pone.0208961. doi:10.1371/journal.pone.0208961
Kasuga T, Bui M, Bernhardt E, Swiecki T, Aram K, Cano LM, Webber J, Brasier C, Press C, Grünwald NJ, et al. Host-induced aneuploidy and phenotypic diversification in the Sudden Oak Death pathogen Phytophthora ramorum. BMC Genomics. 2018;17(1):385. http://dx.doi.org/10.1186/s12864-016-2717-z. doi:10.1186/s12864-016-2717-z
Kasuga T, Hayden KJ, Eyre CA, Croucher PJP, Schechter S, Wright JW, Garbelotto M. Innate resistance and phosphite treatment affect both the pathogen’s and host’s transcriptomes in the tanoak-Phytophthora ramorum pathosystem. Journal of Fungi (Basel, Switzerland). 2021 [accessed 2023 May 16];7(3):198. https://www.mdpi.com/2309-608X/7/3/198. doi:10.3390/jof7030198
Keriö S, Daniels HA, Gómez-Gallego M, Tabima JF, Lenz RR, Søndreli KL, Grünwald NJ, Williams N, Mcdougal R, LeBoldus JM. From genomes to forest management – tackling invasivePhytophthoraspecies in the era of genomics. Canadian journal of plant pathology. Revue Canadienne de Phytopathologie. 2020;42(1):1–29. http://dx.doi.org/10.1080/07060661.2019.1626910. doi:10.1080/07060661.2019.1626910
McCartney MM, Roubtsova TV, Yamaguchi MS, Kasuga T, Ebeler SE, Davis CE, Bostock RM. Effects of Phytophthora ramorum on volatile organic compound emissions of Rhododendron using gas chromatography–mass spectrometry. Analytical and Bioanalytical Chemistry. 2018;410(5):1475–1487. http://dx.doi.org/10.1007/s00216-017-0789-5. doi:10.1007/s00216-017-0789-5
Schweigkofler W, Pastalka T, Abeysekara N, Huffman V, Suslow K. Transmission of the invasive pathogen Phytophthora ramorum from symptomatic to healthy host plants during a five-year period in California. Plant Health Progress. 2022;23(1):33–39. http://dx.doi.org/10.1094/php-06-21-0089-rs. doi:10.1094/php-06-21-0089-rs
Solla A, Moreno G, Malewski T, Jung T, Klisz M, Tkaczyk M, Siebyla M, Pérez A, Cubera E, Hrynyk H, et al. Phosphite spray for the control of oak decline induced by Phytophthora in Europe. Forest Ecology and Management. 2021;485(118938):118938. https://www.sciencedirect.com/science/article/pii/S037811272100027X. doi:10.1016/j.foreco.2021.118938
Tooley PW, Browning M, Vinyard B. Diurnal effects on sporangium and zoospore production by Phytophthora ramorum on Rhododendron “Cunningham’s White.” Mycologia. 2020;112(3):519–532. http://dx.doi.org/10.1080/00275514.2020.1728472. doi:10.1080/00275514.2020.1728472
Yuzon JD, Travadon R, Malar C M, Tripathy S, Rank N, Mehl HK, Rizzo DM, Cobb R, Small C, Tang T, et al. Asexual evolution and forest conditions drive genetic parallelism in Phytophthora ramorum. Microorganisms. 2020 [accessed 2023 May 16];8(6):940. https://www.mdpi.com/2076-2607/8/6/940. doi:10.3390/microorganisms8060940
Zhang B, Zhang Z, Yong S, Yu S, Feng H, Yin M, Ye W, Wang Y, Qiu M. An Oomycete-specific leucine-rich repeat-containing protein is involved in zoospore flagellum development in Phytophthora sojae. Phytopathology. 2022;112(11):2351–2359. http://dx.doi.org/10.1094/PHYTO-12-21-0523-R. doi:10.1094/PHYTO-12-21-0523-R
Zheng K, Lu J, Li J, Yu Y, Zhang J, He Z, Ismail OM, Wu J, Xie X, Li X, et al. Efficiency of chitosan application against Phytophthora infestans and the activation of defense mechanisms in potato. International Journal of Biological Macromolecules. 2021;182:1670–1680. https://www.sciencedirect.com/science/article/pii/S0141813021010722. doi:10.1016/j.ijbiomac.2021.05.097